Thank you for visiting nature.com. You are using a browser version with limited support for CSS. To obtain the best experience, we recommend you use a more up to date browser (or turn off compatibility mode in Internet Explorer). In the meantime, to ensure continued support, we are displaying the site without styles and JavaScript.
Nature Medicine (2023 )Cite this article Various Herbal Formulations

Previous studies showed a low-grade enterovirus infection in the pancreatic islets of patients with newly diagnosed type 1 diabetes (T1D). In the Diabetes Virus Detection (DiViD) Intervention, a phase 2, placebo-controlled, randomized, parallel group, double-blind trial, 96 children and adolescents (aged 6–15 years) with new-onset T1D received antiviral treatment with pleconaril and ribavirin (n = 47) or placebo (n = 49) for 6 months, with the aim of preserving β cell function. The primary endpoint was the mean stimulated C-peptide area under the curve (AUC) 12 months after the initiation of treatment (less than 3 weeks after diagnosis) using a mixed linear model. The model used longitudinal log-transformed serum C-peptide AUCs at baseline, at 3 months, 6 months and 1 year. The primary endpoint was met with the serum C-peptide AUC being higher in the pleconaril and ribavirin treatment group compared to the placebo group at 12 months (average marginal effect = 0.057 in the linear mixed model; 95% confidence interval = 0.004–0.11, P = 0.037). The treatment was well tolerated. The results show that antiviral treatment may preserve residual insulin production in children and adolescent with new-onset T1D. This provides a rationale for further evaluating antiviral strategies in the prevention and treatment of T1D. European Union Drug Regulating Authorities Clinical Trials identifier: 2015-003350-41.
Type 1 diabetes (T1D) is characterized by progressive loss of pancreatic β cell function that leads to lifelong dependence on insulin therapy1. Childhood-onset T1D is a severe disease not only because of disabling acute and late complications leading to shortened life expectancy, but also because of the heavy burden of a demanding therapy regimen on patients and their families2.
The disease is the result of a complex interplay between genetic predisposition, the immune system and environmental factors3,4. However, despite intensive biochemical, immunological, epidemiological and clinical research during the last 100 years, the etiology of T1D remains largely unknown. Some viruses can cause diabetes in animal models5. Viral infections can also contribute to the development of islet autoimmunity in humans6. Enteroviruses in particular have been implicated in the pathogenesis of T1D. Approximately 240 enterovirus types capable of infecting humans have been identified (https://www.picornaviridae.com/); enterovirus infections are common in childhood, ranging from an asymptomatic to a severe clinical presentation7. Meta-analyses showed a clinically significant association between enterovirus infections and the appearance of autoantibodies (T1D stages 1 and 2) and the onset of clinical diabetes (T1D stage 3) (refs. 8,9,10). Enterovirus capsid protein has also been detected in the β cells of patients with T1D11.
In the Diabetes Virus Detection study (DiViD), pancreatic tissue was collected from six adult patients with newly diagnosed T1D12. We demonstrated a low-grade enterovirus infection in the pancreatic islets in all patients and detected infection-competent enteroviruses in the pancreatic tissue from all cases13,14,15. Among known enteroviruses, only some may have diabetogenic properties. Coxsackie B enteroviruses have been associated with T1D in epidemiological studies9. Enteroviruses display tropism to the pancreatic islets because β cells strongly express the receptor used by these viruses16. The possible role of viral infection and the rationale for performing trials targeting enteroviruses were reviewed elsewhere17.
Pleconaril, developed against enteroviruses, clears viruses in β cell models of persistent enterovirus infection18,19,20. Pleconaril significantly reduced mortality due to severe enteroviral sepsis in neonates21. Ribavirin is a nucleoside analog with broad-spectrum antiviral activity against a variety of viruses, including enteroviruses22,23. An in vitro study showed that ribavirin may have an immunomodulatory effect, leading to enhanced interferon-γ (IFNγ) response and thus to a possible additive antiviral effect24.
Despite existing evidence for enteroviral infection initiating the autoimmune response and subsequent β cell destruction in genetically predisposed individuals, it is unclear if the main effect of the viruses is to initiate or drive the disease process, or both25. It is possible that during an acute enteroviral infection the virus spreads to the pancreas, infects β cells and then turns into a terminally deleted, replication-deficient form, which is characteristic of persisting enteroviral infections26. Based on these data, it is possible that eradication of this low-grade infection could improve the pancreas’s ability to secrete insulin after the onset of clinical disease. In this phase 2, randomized, double-blind, placebo-controlled clinical trial (DiViD Intervention), we investigated the effect of antiviral treatment on endogenous insulin production measured using C-peptide in children and adolescents with newly diagnosed T1D.
The trial included a screening period from the day of diagnosis until baseline (up to 3 weeks), a 26-week treatment period and a 26-week off-therapy follow-up period, and an ongoing extended follow-up of 2 additional years (to be reported). Out of 96 randomized participants, 47 (19 females and 28 males) were randomized to pleconaril and ribavirin and 49 (21 females and 28 males) to placebo. Participants were recruited between 20 August 2018 and 20 October 20. Details of the screening, randomization and follow-up of participants are provided in the CONSORT diagram (Fig. 1). Baseline anthropometric, clinical and metabolic characteristics are shown in Table 1.
CONSORT flow diagram for the DiViD interventional trial showing participant flow through each stage of the randomized controlled trial (enrollment, intervention allocation, follow-up and data analysis).
The primary endpoint was endogenous insulin production at 12 months, as assessed by the 2-h serum C-peptide rea under the curve (AUC) during a mixed meal tolerance test (MMTT). Endogenous insulin production was measured at baseline, and then at 3, 6 and 12 months. The primary endpoint was analyzed using a linear mixed model for repeated measures. At 12 months, the serum C-peptide AUC was higher in the pleconaril and ribavirin treatment group compared to the placebo group (average marginal effect (AME) = 0.057 at 12 months in the linear mixed model; 95% confidence interval (CI) = 0.004–0.11, P = 0.037) (Fig. 2a).
a, AUC for C-peptide levels. The box plots report the distribution of AUCs for the C-peptide levels. The numbers beneath the xaxis reflect the number of participants in each group with the respective endpoint at each time point. The line inside the box represents the median; the box boundaries represent the 25th and 75th percentiles (the box is the interquartile range (IQR)); the whiskers represent the highest or lowest values within 1.5× the IQR; the points outside 1.5× the IQR represent the potential outliers, including the maximum and minimum values. The C-peptide levels after 12 months were higher in the pleconaril and ribavirin group compared with the placebo group (AME = 0.057, P = 0.037 from the linear mixed model). b, Peak stimulated C-peptide. The proportion of participants with peak serum C-peptide greater than 0.2 pmol ml−1 at different visits, divided according to antiviral treatment and placebo. *P = 0.04 in the logistic model; risk ratio = 1.29; two-sided 95% CI = 1.03–1.65.
At baseline, the mean (±s.d.) of the non-log-transformed 2-h C-peptide AUC was 0.62 (0.31) pmol ml−1 in the pleconaril and ribavirin group and 0.51 (0.22) pmol ml−1 in the placebo group (P = 0.07). At 12 months, the 2-h C-peptide AUC was 0.55 (0.42) pmol ml−1 in the pleconaril and ribavirin group and 0.39 (0.34) pmol ml−1 in the placebo group. During the 12-month study period, the relative decrease in C-peptide AUC was 11% in the pleconaril and ribavirin group and 24% in the placebo group. Figure 2a shows the AUC boxplots for the C-peptide levels in response to a 2-h MMTT. The observed imbalances in baseline values of the 2-h C-peptide AUC is accounted for by including the baseline values in the linear mixed model for the primary endpoint. Extended Data Fig. 1 shows the individual trajectories of the log-transformed C-peptide AUCs during the study period divided into pleconaril and ribavirin group and placebo group.
At baseline, all participants in both groups had a peak serum C-peptide secretion greater than 0.2 pmol ml−1 measured during MMTT. At 12 months, 36 of 42 participants (86%) in the pleconaril and ribavirin group and 30 of 45 participants (67%) in the placebo group secreted above this cutoff value (P = 0.04 in the logistic model, risk ratio = 1.29, 95% CI = 1.03–1.65) (Fig. 2b).
HbA1c levels were similar in the pleconaril and ribavirin group and in the placebo group both at baseline (88 (18) versus 85 (16) mmol mol−1) and at 12 months (48 (12) versus 51 (9) mmol mol−1) (Table 2). At 3 and 6 months, HbA1c was lower in the pleconaril and ribavirin group compared to the placebo group (P < 0.0001; Table 2and Fig. 3a).
a,b, The box plots show HbA1c (a) and glycated albumin (b), respectively. HbA1c was significantly lower in the pleconaril and ribavirin group compared to the placebo group at 3 and 6 months, but not at 12 months. Glycated albumin was not significantly different between groups at any time point. The numbers beneath the x axis reflect the number of participants in each group with the respective endpoint at each time point. The line inside the box represents the median; the box boundaries represent the 25th and 75th percentiles (the box is the IQR); the whiskers represent the highest or lowest values within 1.5× the IQR; the points outside 1.5× the IQR represent the potential outliers, including the maximum and minimum values.
Glycated albumin was similar in the pleconaril and ribavirin group and the placebo group at baseline and slightly, but not significantly, lower in the treatment group at 3, 6 and 12 months (Table 2 and Fig. 3b).
Insulin dose-adjusted HbA1c (IDAA1c) was similar in the pleconaril and ribavirin group and in the placebo group both at baseline (13.2% versus 12.8%) and at 12 months (9.9% versus 9.9%) (Table 2). At 3 and 6 months, IDAA1c was lower in the pleconaril and ribavirin group compared to the placebo group.
At baseline, the total daily insulin dose per body weight was similar in the pleconaril and ribavirin group and the placebo group (0.75 (0.37) U kg −1day−1 versus 0.71 (0.30) U kg−1 day−1), and the insulin dose was not different between the two groups at any of the follow-up visits (Table 2).
Severe hypoglycemic events, defined as unconsciousness or seizures, were reported in two participants in the placebo group and none in the pleconaril and ribavirin group during the first year of the study (the follow-up part of the study is ongoing). Laboratory analysis of additional secondary endpoints (extended virus analysis and serum proinsulin:C-peptide ratio) will be performed at end of follow-up at 3 years, according to the protocol.
A summary of the key safety events is shown in Table 3. Adverse events were recorded using the Common Terminology Criteria for Adverse Events v.5.0 from the National Institutes of Health. The incidence of adverse events during the first year was 93.6% (44 of 47 participants) in the pleconaril and ribavirin group and 95.9% (47 of 49 participants) in the placebo group. No serious adverse events were reported in either group. Hemolysis is a well-known side effect of ribavirin. Twenty-seven participants in the pleconaril and ribavirin group and 23 in the placebo group had markers of hemolysis in the laboratory analyses at some point across the first year. None had to reduce the dose of ribavirin because of anemia. There were no differences in the occurrence of infections, neither during treatment nor during 6 months after treatment. Two participants in the pleconaril and ribavirin group had a mild coronavirus disease 2019 (COVID-19) infection, 9 and 11 months after baseline (3 and 5 months after ending the treatment period), but none in the placebo group.
In this phase 2 trial involving children and adolescents with newly diagnosed T1D, combination therapy with the antiviral drugs pleconaril and ribavirin for 6 months resulted in a higher endogenous insulin production than placebo 12 months from baseline. The treatment was safe and tolerable.
Viruses contribute to the pathogenesis of T1D, both by damaging β cells or triggering the autoimmune response. Establishment of a low-grade persistent infection in β cells maintains inflammation, leading to the breakdown of tolerance to β cell antigens, β cell dysfunction and stress, each of which might then contribute to autoimmunity as part of a vicious circle17. The antiviral defense mechanisms of β cells are compromised compared to other islet cells27,28. Extensive peptide production by stressed β cells may increase the susceptibility to secreted malformed proteins, which may become antigenic and induce autoimmunity and T1D (stage 2) (refs. 29). This underlying disease mechanism of a low-grade, persistent viral infection may also apply to other autoimmune diseases, that is, cardiomyopathies, celiac disease, multiple sclerosis and Graves’ disease30,31,32,33,34,35.
Beneficial effects of antiviral treatment on β cell function have been observed in the present study at the onset of clinical stage 3 T1D, in a situation where disease progression had been ongoing for months or years. At this late stage of pathogenesis, patients might still have considerable numbers of β cells but they may not function normally36,37. The present study suggests that it could be possible to improve the function of the β cell mass with antiviral treatment. However, antiviral treatment took 12 months to demonstrate a difference in endogenous insulin secretion. This suggests that it may take a long time to experience the effects of antiviral treatment against a persistent, low-grade viral infection. In this study, a positive effect was observed in individuals younger than 15 years, who usually have profound islet inflammation with rapid loss of β cell function preceding diagnosis36. Therefore, it may be more effective to intervene with antivirals at an earlier stage of the disease, that is, stage 2, when there is more β cell function to preserve.
This trial was not designed to distinguish the individual effects of the two study drugs included in this treatment. The main reason for including ribavirin was to prevent the development of viral drug resistance. Ribavirin has a broader antiviral effect both on enteroviruses and several other viruses, and may also have immunomodulatory effects22,23,38. This potential immunomodulatory effect enhances IFNγ, leading to an additive antiviral effect in the context of an in vitro infection24.
The scale of the observed difference in C-peptide secretion between the pleconaril and ribavirin group and the placebo group after 12 months is comparable to the scale of improvement reported for anti-inflammatory medications and lately verapamil, which have been evaluated for newly diagnosed T1D in randomized control trials39,40. However, it is difficult to compare treatment effects across studies because of differences in study design and cohorts. The question around persistence of the observed difference at 12 months will be reported elsewhere, when all participants have been followed for 3 years.
The observed difference in C-peptide AUC at baseline was not significant (P = 0.07). Still, it could cause concern regarding the estimates of the treatment effect from the primary endpoint. For this reason, we adjusted for the baseline in the linear mixed model by including the baseline value among the repeated measurements.
The observed moderate effect of pleconaril and ribavirin may have clinical implications. In general, even a limited preservation of C-peptide levels is associated with improved metabolic control, reduced hypoglycemia and lower long-term microvascular complications41. However, a longer observation period is needed to clarify this issue in participants.
Lower HbA1c was observed in the pleconaril and ribavirin group during the antiviral treatment period (3 and 6 months from baseline) than in the placebo group; at the later time point, 6 months after the end of treatment, the difference in HbA1c between the groups was less pronounced. A known side effect of ribavirin is that it can induce hemolysis, leading to reduced lifespan of erythrocytes and lowering of HbA1c (ref. 42). The frequency and duration of hemolysis was highest in the pleconaril and ribavirin group (Table 3). Thus, we cannot exclude the possibility that this may, at least in part, explain the observed differences in HbA1c at 3 and 6 months. To compensate for the potential reduced lifespan of erythrocytes, we measured glycated albumin. No significant differences in glycated albumin between the groups were observed. Insulin doses changed as usual during the first year of insulin treatment, showing no significant differences between the treatment and placebo groups.
The trial has some limitations. First, the study included a small number of participants. The statistical power calculation for this trial was aimed at the primary endpoint and was not designed to estimate the power to detect secondary endpoints, which have greater between-person variation and laboratory analytical variation. Effects beyond those in the population studied, that is, centers, age, sex and potential role of COVID-19, could not be credibly examined. Second, the observed significant difference between the treatment and placebo group should be replicated using a higher number of participants of different ages and living in different countries, thus representing different microbiological environments. Third, information regarding continuous glucose monitoring was not available for all participants. Possible persisting low-grade enterovirus infection in the pancreas is difficult to detect from serum or samples taken from the periphery, even if the virus is detected in the pancreatic islets15. Because all trial participants were negative for enterovirus RNA using a sensitive PCR with reverse transcription (RT–PCR) in serum, stool and respiratory samples, we could not correlate the efficacy of treatment with the possible presence of such an infection.
In conclusion, this study shows that among children and adolescents with newly diagnosed T1D, the combination therapy of two antiviral drugs, pleconaril and ribavirin, resulted in higher residual endogenous insulin production than placebo. These results provide a rationale for future studies to evaluate the efficacy of antiviral drugs in the prevention and treatment of T1D.
We conducted this phase 2, randomized, placebo-controlled, double-blind, parallel-group clinical trial at two sites: Oslo University Hospital, Norway and Steno Diabetes Center Copenhagen/Herlev University Hospital, Copenhagen, Denmark. Participants were children and adolescents aged 6–15 years with newly diagnosed T1D, randomly assigned in a 1:1 ratio to receive either a combination of pleconaril and ribavirin or placebo. We used block randomization, stratified according to site. The trial included a screening period from the day of diagnosis until baseline (up to 3 weeks), a 26-week treatment period and a 26-week off-therapy follow-up period, as well as an ongoing extended follow-up of two additional years (to be reported). The trial was conducted in accordance with the principles of the Declaration of Helsinki 2013, the International Conference on Harmonization Good Clinical Practice guidelines and applicable regulatory requirements. Approvals were obtained from the governmental and regional research ethics committees in Oslo and Copenhagen. Written informed consent was obtained from the participant’s caregiver and participants gave their verbal consent after receiving age-adjusted information. For the complete study protocol, see the Supplementary Information.
Participants were aged between 6 and 15 years, had received a diagnosis of stage 3 T1D according to the American Diabetes Association criteria8. They were recruited from pediatric departments in southern Norway and in the Copenhagen Capital Region, Denmark between August 2018 and October 2020; they were able to undergo randomization within 3 weeks after the first insulin injection. Randomization was performed by statisticians at the Clinical Trial Support Unit in Oslo, having no contact with the trial personnel, and separate from the study centers. Masking success was ensured by the appointed good clinical practice (GCP) monitors.
The main inclusion criteria, which had to be fulfilled at screening before receiving the study agent, were: (1) diagnosed with T1D (International Statistical Classification of Diseases and Related Health Problems, 10th Revision code E10.9) with the first injection of insulin 3 weeks before inclusion; (2) willing and capable of taking the study drugs and meeting for tests and follow-up as described; (3) providing signed informed consent and expected to cooperate for the treatment and follow-up obtained and documented according to the International Council for Harmonisation of Technical Requirements for Pharmaceuticals for Human Use GCP, as well as national and local regulations; and (4) aged from 6.00 to 15.99 years at inclusion.
The main exclusion criteria were: (1) treatment with any oral or injected antidiabetic medication other than insulin; (2) a history of hemolytic anemia or significantly abnormal hematology results at screening; (3) history of severe cardiac disease in the previous 6 months; (4) impaired renal function; (5) participation in other clinical trials with a new chemical entity within the previous 3 months; (6) inability or unwillingness to comply with the provisions of the study protocol; (7) females who were lactating or pregnant; (8) males or females (after menarche) not willing to use highly effective contraception (progesterone-only hormonal anticonception with inhibition of ovulation or sexual abstinence) and barrier contraception (condoms), if sexually active during the treatment period and in the following 7 months; (9) presence of a serious disease or condition, which in the opinion of the investigators made the patient ineligible for the study.
Pleconaril and ribavirin were administered as oral solutions (to enable weight-based dosing) at home as separate mixtures for 26 weeks. Combination treatment was chosen to increase and broaden the antiviral effect and to reduce the risk of emergence of drug-resistant virus variants. A 6-month treatment was chosen based on clinical experience from treating other chronic viral infections. In cell models, pleconaril eradicated persistent enterovirus infection in 5–6 weeks20. The pleconaril dose was 5 mg kg−1 twice daily. The maximum daily dose was 600 mg. The ribavirin dose was 7.5 mg kg−1 twice daily. The maximum daily dose was 1,000 mg if body weight was less than 75 kg and 1,200 mg if body weight was more than 75 kg. Study drugs were delivered by Apodemus AB. Investigators and participants were blinded to the study drugs and placebo. Drugs or placebo were distributed to patients at the study visits to be administered at home and taken twice daily together with food. For details, see the Supplementary Information. Compliance was assessed by interviewing participants and care providers, and by measuring the returned drug bottles at each study visit.
The primary endpoint was endogenous insulin production, as assessed according to the 2-h serum C-peptide AUC during an MMTT at 12 months. The AUC was calculated at each visit using the trapezoidal rule on five measurements collected during the 2-h test (at 0, 15, 30, 60 and 90 min, respectively). Secondary endpoints included a preserved peak C-peptide level greater than 0.2 pmol ml−1 during MMTT, insulin dosage, HbA1c, glycated albumin and severe hypoglycemic events.
All laboratory analyses were performed with the same methods throughout the trial for all participants. All sampling and biobanking followed the INNODIA Master Protocol and Standard of Procedures43. MMTT was performed in the morning at the hospital in the fasting state with blood sampling at baseline and 15, 30, 60, 90 and 120 min after ingestion of a standardized liquid meal (Ensure Plus or equivalent, 6 ml kg−1 bodyweight, maximum 360 ml)44. For participants with missing time points during the MMTT, a weighted average of the available time points was used.
HbA1c was analyzed using international standard methods (coefficient of variantion (CV) 2%). Glycated albumin (%) was determined with liquid chromatography–tandem mass spectrometry (CV 2–6%) (ref. 45). Safety-related laboratory tests were performed using standard hospital assays.
Enterovirus RT–PCR was performed using nasal swab, nasopharyngeal aspirates, saliva, stool and serum samples as described previously46.
The sample size calculation is based on the efficacy continuous variable ‘2-h AUC C-peptide’. Because this variable is strongly skewed to the right, a logarithmic transformation (log(x + 1)) is used. By using X data from large studies47,48,49 and claiming an 85% test power to detect a 50% absolute improvement with a 5% significance in the treatment group compared to the placebo group (mean = 0.179, s.d. = 0.172) after 12 months45. Eighty-six patients must be included in each group. This sample size calculation (n = 172) was based on an independent samples t-test when comparing 2-h AUC C-peptide values after 1 year. A recent publication showed that when using analysis of covariance, adjusting for baseline ‘AUC C-peptide’ and age, instead of a t-test, sample size may be reduced by 50% (ref. 47). As we expected a dropout rate of 10%, we decided to include 96 (86 + 10) patients in the study.
Both efficacy and safety analyses included all participants who had received at least one dose of pleconaril and ribavirin or placebo.
The primary null hypothesis was evaluated in the full analysis set (FAS), defined as all participants fulfilling the entry criteria who were randomly assigned to a treatment group. Sensitivity analysis was performed in the per protocol analysis set, which included all participants in the FAS who met the study eligibility criteria, with no major protocol deviations affecting treatment efficacy, and who complied with the prescribed treatment. Safety data were analyzed for all participants having taken the study medication. Individuals who withdrew from the study were included in the safety analysis. The primary endpoint was analyzed using a linear mixed model for repeated measures that was fitted on the log-transformed C-peptide AUC data. The model used the longitudinal log-transformed serum C-peptide AUCs at baseline, 3 months, 6 months and 1 year as the response variable and included categorical effects of site, treatment, time and treatment-by-time interaction, as well as a random effect for the patient ID to account for repeated measurements. The linear mixed model included all repeated measurements of C-peptide AUCs, which were available for 89 participants (42 in the pleconaril and ribavirin group and 47 in the placebo group, of which 87 had the 12-month measurement and the remaining 2 only had the previous measurements). The treatment effect was defined as the AME; the primary estimate of treatment effect was obtained as the AME at 12 months50.
The detailed statistical analysis plan (SAP) was produced before the final database lock, before extracting files from the INNODIA clinical database and before unblinding of treatment allocation. The SAP was signed by the authorized chief statistician of Oslo University Hospitals.
We fitted a linear mixed model on the mean residual insulin secretion, using the values collected at baseline, 3 months, 6 months and 12 months as a longitudinal outcome variable, and including a random effect for patient ID to account for dependency among measurements taken for the same individual. We included categorical effects for the study site, treatment, time and an interaction term between treatment and time. Because baseline values were collected before randomization, the treatment effect was set to 0 at baseline. Including the baseline value as one of the repeated measurements and forcing the treatment effect to be null at baseline is not only equivalent to adjusting for baseline, but is also the preferred alternative to analyze longitudinal endpoints51,52.
The strength of the model we used lies in the fact that we also included the measurements taken at 3 and 6 months, which provides additional power for the analysis. Using exclusively the change from baseline to 12 months would have resulted in less power. The present study had 96 participants; only 87 had records at 12 months. Eighty-nine patients had records at 3 or 6 months; such information can be used to strengthen the conclusion of the analyses. The measurements at 3 and 6 months were listed in the protocol as secondary endpoints; we analyzed them simultaneously in the proposed model. To ensure that we did not incur issues of multiplicity, we defined the primary treatment effect as the treatment effect at 12 months, which is consistent with the protocol. Including the interaction between time and treatment in the model allows us to do so by defining the treatment effect at 12 months as the AME at 12 months.
The use of multiple measurements reduces the uncertainty and increases the power of the findings, thus resulting in a statistically significant (P = 0.037) effect of the treatment at 12 months, while simultaneously preserving the adjustment for baseline and being equivalent to analyzing the change from baseline values.
To investigate the robustness of the results, we also ran a Wilcoxon t-test on the change from baseline to 12 months without including other repeated measurements. Although such a test results in a non-significant (P = 0.19) effect, it confirms the same trend toward efficacy and a similar estimate of the treatment effect as found by the linear mixed model. The loss of significance is most probably due to the reduced power when using only the 12-month measurements. The increased power and the ability to adjust for baseline values are at the basis of our model choice for the primary analysis. Nevertheless, this, and the other sensitivity analyses we conducted, all pointed in the same direction, although not all of them showed a significant effect. This pattern was consistent with the level of significance observed in the primary analysis (0.04) and confirmed the need for a larger follow-up study to confirm these findings.
The secondary endpoints of mean insulin dosage per body weight, HbA1c, glycated albumin and insulin dose-adjusted HbA1c, were analyzed using a linear mixed model for repeated measures with the same structure and covariates as for the primary endpoint.
The dichotomous secondary endpoint corresponding to the proportion of patients with peak C-peptide above 0.2 pmol ml−1 at 12 months was analyzed with a logistic model including site and categorical treatment as covariates. Missing data for all continuous missing outcomes were assumed to be missing at random and were imputed implicitly using a mixed model for repeated measures.
Results are reported as the mean (s.d.) for continuous outcomes and n (%) for binary outcomes. All reported P values are two-sided.
A safety and data monitoring committee was established before starting participant recruitment (committees members are listed in the ‘Acknowledgements’).
The trial was monitored closely with regard to safety. The data monitoring committee assessed the safety data and adverse events through the course of the trial. Efficacy data (primary endpoint) were not analyzed before unblinding. Therefore, no interim analysis was performed on the primary endpoint and no adaptive design or error rate control was needed.
Further information on research design is available in the Nature Portfolio Reporting Summary linked to this article.
Data collected for the study and presented herein will be made available to others when the end-of-trial reports, after 3 years of follow-up, have been published. Anonymized participant data can be obtained upon reasonable request from the corresponding author. Proposals will be reviewed on the basis of scientific merit, ethical review, available resources and regulatory requirements. After approval of a proposal, anonymized data will be made available for reuse. The corresponding author has the right to review and comment on any draft papers based on these data before publication. Availability will follow General Data Protection Regulations. Data will be organized in a data dictionary and participant data will be de-identified. Related study documents, including the informed consent forms (in Norwegian) will also be available. The study protocols and the SAP are found in the Supplementary Information. All data requests should be sent to the corresponding author (knut.dahl-jorgensen@medisin.uio.no).
All the code used for the descriptive tables and the analysis of the primary and safety endpoints is publicly available at https://github.com/ericaponzi/DiViDInt-R-code.
Chiang, J. L., Kirkman, M. S., Laffel, L. M. & Peters, A. L. Type 1 diabetes through the life span: a position statement of the American Diabetes Association. Diabetes Care 37, 2034–2054 (2014).
Article PubMed PubMed Central Google Scholar
Rawshani, A. et al. Excess mortality and cardiovascular disease in young adults with type 1 diabetes in relation to age at onset: a nationwide, register-based cohort study. Lancet 392, 477–486 (2018).
Article PubMed PubMed Central Google Scholar
Atkinson, M. A., von Herrath, M., Powers, A. C. & Clare-Salzler, M. Current concepts on the pathogenesis of type 1 diabetes—considerations for attempts to prevent and reverse the disease. Diabetes Care 38, 979–988 (2015).
Article CAS PubMed PubMed Central Google Scholar
Hober, D. & Sauter, P. Pathogenesis of type 1 diabetes mellitus: interplay between enterovirus and host. Nat. Rev. Endocrinol. 6, 279–289 (2010).
Jun, H. S. & Yoon, J. W. The role of viruses in type I diabetes: two distinct cellular and molecular pathogenic mechanisms of virus-induced diabetes in animals. Diabetologia 44, 271–285 (2001).
Article CAS PubMed Google Scholar
Vehik, K. et al. Prospective virome analyses in young children at increased genetic risk for type 1 diabetes. Nat. Med. 25, 1865–1872 (2019).
Article CAS PubMed PubMed Central Google Scholar
de Crom, S. C., Rossen, J. W., van Furth, A. M. & Obihara, C. C. Enterovirus and parechovirus infection in children: a brief overview. Eur. J. Pediatr. 175, 1023–1029 (2016).
Article CAS PubMed PubMed Central Google Scholar
Insel, R. A. et al. Staging presymptomatic type 1 diabetes: a scientific statement of JDRF, the Endocrine Society, and the American Diabetes Association. Diabetes Care 38, 1964–1974 (2015).
Article CAS PubMed PubMed Central Google Scholar
Isaacs, S. R. et al. Enteroviruses and risk of islet autoimmunity or type 1 diabetes: systematic review and meta-analysis of controlled observational studies detecting viral nucleic acids and proteins. Lancet Diabetes Endocrinol. 11, 578–592 (2023).
Article CAS PubMed Google Scholar
Faulkner, C. L. et al. The virome in early life and childhood and development of islet autoimmunity and type 1 diabetes: a systematic review and meta-analysis of observational studies. Rev. Med. Virol. 31, 1–14 (2021).
Article CAS PubMed Google Scholar
Richardson, S. J., Leete, P., Bone, A. J., Foulis, A. K. & Morgan, N. G. Expression of the enteroviral capsid protein VP1 in the islet cells of patients with type 1 diabetes is associated with induction of protein kinase R and downregulation of Mcl-1. Diabetologia 56, 185–193 (2013).
Article CAS PubMed Google Scholar
Krogvold, L. et al. Pancreatic biopsy by minimal tail resection in live adult patients at the onset of type 1 diabetes: experiences from the DiViD study. Diabetologia 57, 841–843 (2014).
Krogvold, L. et al. Live enteroviruses, but not other viruses, detected in human pancreas at the onset of type 1 diabetes in the DiViD study. Diabetologia 65, 2108–2120 (2022).
Article CAS PubMed PubMed Central Google Scholar
Krogvold, L. et al. Detection of a low-grade enteroviral infection in the islets of Langerhans of living patients newly diagnosed with type 1 diabetes. Diabetes 64, 1682–1687 (2015).
Article CAS PubMed Google Scholar
Oikarinen, S. et al. Characterisation of enterovirus RNA detected in the pancreas and other specimens of live patients with newly diagnosed type 1 diabetes in the DiViD study. Diabetologia 64, 2491–2501 (2021).
Article CAS PubMed PubMed Central Google Scholar
Ifie, E. et al. Unexpected subcellular distribution of a specific isoform of the Coxsackie and adenovirus receptor, CAR-SIV, in human pancreatic beta cells. Diabetologia 61, 2344–2355 (2018).
Article CAS PubMed PubMed Central Google Scholar
Dunne, J. L. et al. Rationale for enteroviral vaccination and antiviral therapies in human type 1 diabetes. Diabetologia 62, 744–753 (2019).
Article CAS PubMed PubMed Central Google Scholar
Berg, A.-K., Olsson, A., Korsgren, O. & Frisk, G. Antiviral treatment of Coxsackie B virus infection in human pancreatic islets. Antiviral Res. 74, 65–71 (2007).
Article CAS PubMed Google Scholar
Hayden, F. G. et al. Oral pleconaril treatment of picornavirus-associated viral respiratory illness in adults: efficacy and tolerability in phase II clinical trials. Antivir. Ther. 7, 53–65 (2002).
Article CAS PubMed Google Scholar
Honkimaa, A. et al. Eradication of persistent coxsackievirus B infection from a pancreatic cell line with clinically used antiviral drugs. J. Clin. Virol. 128, 104334 (2020).
Article CAS PubMed Google Scholar
Abzug, M. J. et al. A randomized, double-blind, placebo-controlled trial of pleconaril for the treatment of neonates with enterovirus sepsis. J. Pediatric. Infect. Dis. Soc. 5, 53–62 (2016).
Sioofy-Khojine, A. B., Honkimaa, A. & Hyöty, H. A preclinical assessment to repurpose drugs to target type 1 diabetes-associated type B coxsackieviruses. Diabet. Med. 37, 1849–1853 (2020).
Article CAS PubMed Google Scholar
Kirsi, J. J. et al. Broad-spectrum antiviral activity of 2-beta-d -ribofuranosylselenazole-4-carboxamide, a new antiviral agent. Antimicrob. Agents Chemother. 24, 353–361 (1983).
Article CAS PubMed PubMed Central Google Scholar
Kupke, P. et al. Immunomodulation of natural killer cell function by ribavirin involves TYK-2 activation and subsequent increased IFN-γ secretion in the context of in vitro hepatitis E virus infection. Cells 12, 453 (2023).
Article CAS PubMed PubMed Central Google Scholar
Geravandi, S., Liu, H. & Maedler, K. Enteroviruses and T1D: is it the virus, the genes or both which cause T1D. Microorganisms 8, 1017 (2020).
Article CAS PubMed PubMed Central Google Scholar
Chapman, N. M. Persistent enterovirus infection: little deletions, long infections. Vaccines 10, 770 (2022).
Article CAS PubMed PubMed Central Google Scholar
Op de Beeck, A. & Eizirik, D. L. Viral infections in type 1 diabetes mellitus—why the β cells? Nat. Rev. Endocrinol. 12, 263–273 (2016).
Article CAS PubMed Google Scholar
Marroqui, L. et al. Differential cell autonomous responses determine the outcome of coxsackievirus infections in murine pancreatic α and β cells. eLife 4, e06990 (2015).
Article PubMed PubMed Central Google Scholar
Eizirik, D. L., Miani, M. & Cardozo, A. K. Signalling danger: endoplasmic reticulum stress and the unfolded protein response in pancreatic islet inflammation. Diabetologia 56, 234–241 (2013).
Article CAS PubMed Google Scholar
Bouin, A. et al. Enterovirus persistence in cardiac cells of patients with idiopathic dilated cardiomyopathy is linked to 5′ terminal genomic RNA-deleted viral populations with viral-encoded proteinase activities. Circulation 139, 2326–2338 (2019).
Article CAS PubMed PubMed Central Google Scholar
Kahrs, C. R. et al. Enterovirus as trigger of coeliac disease: nested case-control study within prospective birth cohort. BMJ 364, l231 (2019).
Article PubMed PubMed Central Google Scholar
Lindfors, K. et al. Metagenomics of the faecal virome indicate a cumulative effect of enterovirus and gluten amount on the risk of coeliac disease autoimmunity in genetically at risk children: the TEDDY study. Gut 69, 1416–1422 (2020).
Article CAS PubMed Google Scholar
Oikarinen, M. et al. Enterovirus infections are associated with the development of celiac disease in a birth cohort study. Front. Immunol. 11, 604529 (2021).
Article PubMed PubMed Central Google Scholar
Robinson, W. H. & Steinman, L. Epstein–Barr virus and multiple sclerosis. Science 375, 264–265 (2022).
Article CAS PubMed Google Scholar
Hammerstad, S. S. et al. Detection of enterovirus in the thyroid tissue of patients with Graves’ disease. J. Med. Virol. 85, 512–518 (2013).
Article CAS PubMed Google Scholar
Krogvold, L. et al. Function of isolated pancreatic islets from patients at onset of type 1 diabetes: insulin secretion can be restored after some days in a non-diabetogenic environment in vitro: results from the DiViD study. Diabetes 64, 2506–2512 (2015).
Article CAS PubMed Google Scholar
In’t Veld, P. Insulitis in human type 1 diabetes: a comparison between patients and animal models. Semin. Immunopathol. 36, 569–579 (2014).
Article PubMed PubMed Central Google Scholar
Mondelli, M. U. The multifaceted functions of ribavirin: antiviral, immunomodulator, or both? Hepatology 60, 1126–1129 (2014).
Article CAS PubMed Google Scholar
Jacobsen, L. M. et al. Comparing beta cell preservation across clinical trials in recent-onset type 1 diabetes. Diabetes Technol. Ther. 22, 948–953 (2020).
Article CAS PubMed PubMed Central Google Scholar
Forlenza, G. P. et al. Effect of verapamil on pancreatic beta cell function in newly diagnosed pediatric type 1 diabetes: a randomized clinical trial. JAMA 329, 990–999 (2023).
Article CAS PubMed PubMed Central Google Scholar
Jeyam, A. et al. Clinical impact of residual C-peptide secretion in type 1 diabetes on glycemia and microvascular complications. Diabetes Care 44, 390–398 (2021).
Article CAS PubMed Google Scholar
Bodenheimer, H. C.Jr et al. Tolerance and efficacy of oral ribavirin treatment of chronic hepatitis C: a multicenter trial. Hepatology 26, 437–477 (1997).
Dunger, D. B. et al. INNODIA Master Protocol for the evaluation of investigational medicinal products in children, adolescents and adults with newly diagnosed type 1 diabetes. Trials 23, 414 (2022).
Article PubMed PubMed Central Google Scholar
Greenbaum, C. J. et al. Mixed-meal tolerance test versus glucagon stimulation test for the assessment of β-cell function in therapeutic trials in type 1 diabetes. Diabetes Care 31, 1966–1971 (2008).
Article PubMed PubMed Central Google Scholar
Brede, C., Hop, B., Jørgensen, K. & Skadberg, Ø. Measurement of glycated albumin in serum and plasma by LC–MS/MS. Scand. J. Clin. Lab. Invest. 76, 195–201 (2016).
Article CAS PubMed Google Scholar
Honkanen, H. et al. Human enterovirus 71 strains in the background population and in hospital patients in Finland. J. Clin. Virol. 56, 348–353 (2013).
Article CAS PubMed Google Scholar
Palmer, J. P. et al. C-peptide is the appropriate outcome measure for type 1 diabetes clinical trials to preserve β-cell function: report of an ADA workshop, 21–22 October 2001. Diabetes 53, 250–264 (2004).
Article CAS PubMed Google Scholar
Ludvigsson, J. et al.GAD treatment and insulin secretion in recent-onset type 1 diabetes.N. Engl.J. Med.359, 1909–1920 (2008).
Article CAS PubMed Google Scholar
Bundy, B. N. & Krischer, J. P. A model-based approach to sample size estimation in recent onset type 1 diabetes. Diabetes Metab. Res. Rev. 32, 827–834 (2016).
Article CAS PubMed PubMed Central Google Scholar
Searle, S. R., Speed, F. M. & Milliken, G. A. Population marginal means in the linear model: an alternative to least squares means. Am. Stat. 34, 216–221 (1980).
Coffman, C. J., Edelman, D. & Woolson, R. F. To condition or not condition? Analysing ‘change’ in longitudinal randomised controlled trials. BMJ Open 6, e013096 (2016).
Article PubMed PubMed Central Google Scholar
Twisk, J. W. R. Analysis of Data from Randomized Controlled Trials: a Practical Guide Chap. 3 (Springer, 2021).
We thank the participants and their families for their tremendous efforts during the COVID-19 pandemic lockdowns. We thank our colleagues at the local pediatric departments in Norway for their help recruiting the participants for the trial. We thank the study nurses T. Roald and C. Steinhovden, Oslo University Hospital, and A. Hillersborg, Herlev University Hospital. We thank L. Sandvik who performed the statistical power calculations. This trial is associated with the INNODIA Consortium: we thank C. Matthieu and the late D. Dunger for their support; P. Jaroslaw Chmura and S. Brunak, University of Copenhagen for managing the INNODIA database and electronic case record forms (CRFs) used in the trial; S. Bruggraber, University of Cambridge for practical support and advice regarding the shipment of samples and biobanking; M. Knip, University of Helsinki for performing the autoantibody analysis; C. Brede and Ø. Skaberg, Stavanger University Hospital, for performing the analysis of the glycated albumin; J. P. Berg, University of Oslo for mentoring I.M.M.; the Data Safety Monitoring Committee (T. J. Berg (leader), University of Oslo, R. Hanås, Uddevalla Hospital, Sweden, M. LeBlanc, Statistician, Oslo University Hospital and M. Lagging, University of Gothenburg); J. R. Larsen, Oslo University Hospital for support with study planning; the Clinical Trial Support Unit, Oslo University Hospital (M. Colban for assisting with the applications to the Norwegian Medicines Agency, B. L. Adamsen for the GCP monitoring of the trial, and I. Christoffer Olsen and M. Valberg for supervising the statistical analysis); B. Sonne Rasmussen for GCP monitoring in Copenhagen; and A. Mander, University of Cardiff for advice regarding the SAP. This study was funded by the Health Region South East, Norway (grant no. 2016119); the Juvenile Diabetes Research Foundation (grant no. 2-SRA-2019-810-M-B); and European Union supported by IMI2-JU under grant agreement no. 115797 (INNODIA) and no. 948268 (INNODIA HARVEST). This joint undertaking received support from the Union’s Horizon 2020 research and innovation program and through the EFPIA, JDRF and The Leona M. and Harry B. Helmsley Charitable Trust. All grants were provided to the principal investigator K.D.-J. The funders of the study had no role in study design, data collection, data analysis, data interpretation or writing of the manuscript.
These authors contributed equally: Lars Krogvold, Ida Maria Mynarek.
Division of Paediatric and Adolescent Medicine, Oslo University Hospital, Oslo, Norway
Lars Krogvold, Ida Maria Mynarek, Trine Roald & Knut Dahl-Jørgensen
Clinical Trial Unit, Oslo University Hospital, Oslo, Norway
Steno Diabetes Center Copenhagen, Herlev University Hospital, Copenhagen, Denmark
Freja Barrett Mørk, Trine Witzner Hessel & Jesper Johannesen
Department of Clinical Medicine, Faculty of Health and Medical Sciences, University of Copenhagen, Copenhagen, Denmark
Nina Lindblom & Jacob Westman
National Institute for Health and Care Research Cambridge Biomedical Research Centre, Core Biochemistry Assay Laboratory, Cambridge, UK
Faculty of Medicine and Health Technology, Tampere University, Tampere, Finland
Linköping University, Linköping, Sweden
Faculty of Medicine, University of Oslo, Oslo, Norway
Kristian F. Hanssen & Knut Dahl-Jørgensen
You can also search for this author in PubMed Google Scholar
You can also search for this author in PubMed Google Scholar
You can also search for this author in PubMed Google Scholar
You can also search for this author in PubMed Google Scholar
You can also search for this author in PubMed Google Scholar
You can also search for this author in PubMed Google Scholar
You can also search for this author in PubMed Google Scholar
You can also search for this author in PubMed Google Scholar
You can also search for this author in PubMed Google Scholar
You can also search for this author in PubMed Google Scholar
You can also search for this author in PubMed Google Scholar
You can also search for this author in PubMed Google Scholar
You can also search for this author in PubMed Google Scholar
You can also search for this author in PubMed Google Scholar
K.D.-J. is the principal investigator of the DiViD studies, including this intervention trial. He conceived the project, drafted the protocol, organized the staff and collaborations with partners, partnered with Apodemus/Curovir AB for the trial, organized the applications to the medicine agencies and ethics committees, organized the funding and the collaboration with INNODIA and related laboratories, planned and organized the statistical analysis and wrote the first and final drafts of this manuscript. L.K. managed the trial together with K.D.-J. and supervised the staff. He actively participated in writing the protocols, the applications to the medicines agencies, ethics committees and funding agencies, organized the collaboration with INNODIA database staff and the development of the INNODIA electronic CRF system. He participated in writing the SAP, analyzed the data and wrote all the drafts of the manuscript. I.M.M. was a PhD candidate and physician and performed all the study visits for the participants in Oslo, entered clinical data into the CRF system, collected and prepared all the samples together with T.R. and managed the biobank. She ensured the quality of the database, participated in writing the SAP, analyzed the data and wrote the manuscript. E.P. was a statistician. She participated in writing the SAP, analyzed the data and wrote the first and final drafts of the manuscript. F.B.M. and T.W.H. were PhD candidates and physicians and performed all the study visits in Copenhagen. They entered clinical data into the CRF system, and collected and prepared all the samples for storing and shipping. T.R. was a study nurse and performed all the study visits of participants in Oslo, entered clinical data into the CRF system and collected and prepared all the samples together with I.M.M. She organized the biobank and the shipping of samples to the international laboratories. J.W. was responsible for managing the GMP production of pleconaril and ribavirin at Anthem BioSciences in Bangalor and the GMP production of the investigational medicinal product at Unimedic in Matfors, Sweden. He also prepared the investigator’s brochure and investigational medicinal product dossier and arranged the logistics and distribution of the drugs with Birka BioStorages. N.L. participated in planning the trial. She actively participated in writing the protocol, the applications to the medicines agencies and ethics committees and helped writing the final manuscript. P.B. and the National Institute for Health and Care Research Cambridge Biomedical Research Centre, Core Biochemistry Assay Laboratory, Cambridge University Hospitals NHS Foundation Trust analyzed the serum C-peptide samples. H.H. participated in the planning of the trial, tested the potential antiviral drugs, analyzed all virus data for the trial and assisted with preparing the final manuscript. J.L. participated in planning the trial, contributed to the C-peptide data with the statistical power calculation and contributed to writing the final manuscript. K.F.H. participated in trial planning and in writing the final manuscript. J.J. was the trial manager in Copenhagen and supervised the staff. He applied to the Danish ethics committee and the Danish medicines agency. He participated in the interpretation of the results and helped with preparing the final manuscript. All authors collected the data, contributed intellectually and critically reviewed the scientific content of the manuscript. All authors approved the final manuscript as submitted and agreed to be accountable for all aspects of the work. L.K. and K.D.-J. are the guarantors of this work and, as such, had full access to all the data in the study, verified the data and take responsibility for the integrity of the data and the accuracy of the data analysis. All authors had full access to all the data in the study and had final responsibility for the decision to submit for publication.
Correspondence to Knut Dahl-Jørgensen.
H.H. is a shareholder and member of the board of Vactech Ltd, which develops vaccines against picornaviruses. N.L. and J.W. are employees of Apodemus/Curovir AB, which own the rights to the commercialization of the results of this trial. Curovir AB is developing antiviral drugs for enterovirus-induced diseases. The other authors declare no competing interests.
Nature Medicine thanks Richard Oram, Luke Ouma and the other, anonymous, reviewer(s) for their contribution to the peer review of this work. Primary Handling Editors: Sonia Muliyil and Jennifer Sargent, in collaboration with the Nature Medicine team.
Publisher’s note Springer Nature remains neutral with regard to jurisdictional claims in published maps and institutional affiliations.
Longitudinal plots with trajectories of log transformed C-peptide AUC, all participants, divided into treatment and placebo group. N = 91 (47 in placebo and 44 in active group).
Supplementary treatment description, Tables 1 and 2 and legend to Extended Data Fig. 1.
Open Access This article is licensed under a Creative Commons Attribution 4.0 International License, which permits use, sharing, adaptation, distribution and reproduction in any medium or format, as long as you give appropriate credit to the original author(s) and the source, provide a link to the Creative Commons license, and indicate if changes were made. The images or other third party material in this article are included in the article’s Creative Commons license, unless indicated otherwise in a credit line to the material. If material is not included in the article’s Creative Commons license and your intended use is not permitted by statutory regulation or exceeds the permitted use, you will need to obtain permission directly from the copyright holder. To view a copy of this license, visit http://creativecommons.org/licenses/by/4.0/.
Krogvold, L., Mynarek, I.M., Ponzi, E. et al. Pleconaril and ribavirin in new-onset type 1 diabetes: a phase 2 randomized trial. Nat Med (2023). https://doi.org/10.1038/s41591-023-02576-1
DOI: https://doi.org/10.1038/s41591-023-02576-1
Anyone you share the following link with will be able to read this content:
Sorry, a shareable link is not currently available for this article.
Provided by the Springer Nature SharedIt content-sharing initiative
Nature Medicine (Nat Med) ISSN 1546-170X (online) ISSN 1078-8956 (print)
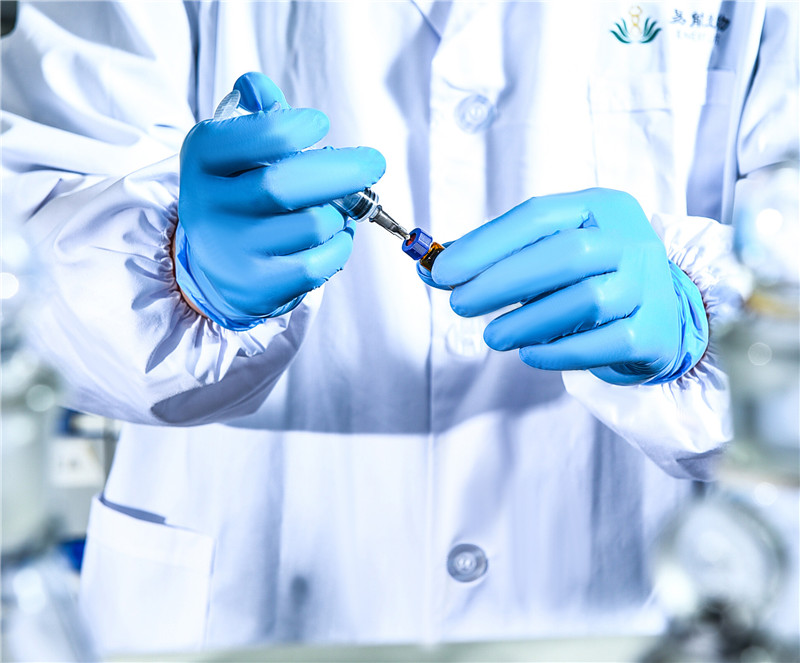
Gc Testing Sign up for the Nature Briefing newsletter — what matters in science, free to your inbox daily.